With the intensifying competition in the beverage packaging market, reducing the thickness of materials, minimizing wall thickness, reducing costs, improving material utilization, facilitating recycling and convenience of use have become important goals for many can manufacturing companies.
One of the key aspects of cost reduction in the production of aluminum cans is reducing the thickness of the aluminum strip used for making the cans. The thickness of can body materials has decreased from 0.42mm in the 1970s to the current 0.254mm (most are still around 0.28mm), resulting in a 39.5% reduction over the past 30 years. For every 0.01mm reduction in can body thickness, the material cost can be saved by $0.22 per thousand cans. Over the decades, the manufacturing technology of aluminum cans has been continuously improved, leading to a significant reduction in the weight of aluminum cans. In the early 1960s, the weight of a thousand aluminum cans (including can bodies and lids) was 55 pounds (approximately 25 kilograms). By the mid-1970s, it had dropped to 44.81 pounds, further reduced to 33 pounds in the late 1990s, and now it is below 30 pounds, nearly halving the weight compared to 40 years ago. Since the 1980s, canning companies in the United States have made breakthroughs in sealing machinery and other technologies, resulting in a noticeable decrease in the thickness of aluminum used in aluminum cans, from 0.343mm to around 0.259mm.
There has also been significant progress in lightweighting can lids. The thickness of the aluminum used for can lids has decreased from 0.39mm to 0.24mm. The diameter of the lids has also been reduced, leading to a continuous reduction in lid weight. At the same time, the canning speed has increased significantly. In the 1970s, the production rate was only 650-1000 cans per minute, but now it has reached over 2000 cans per minute.
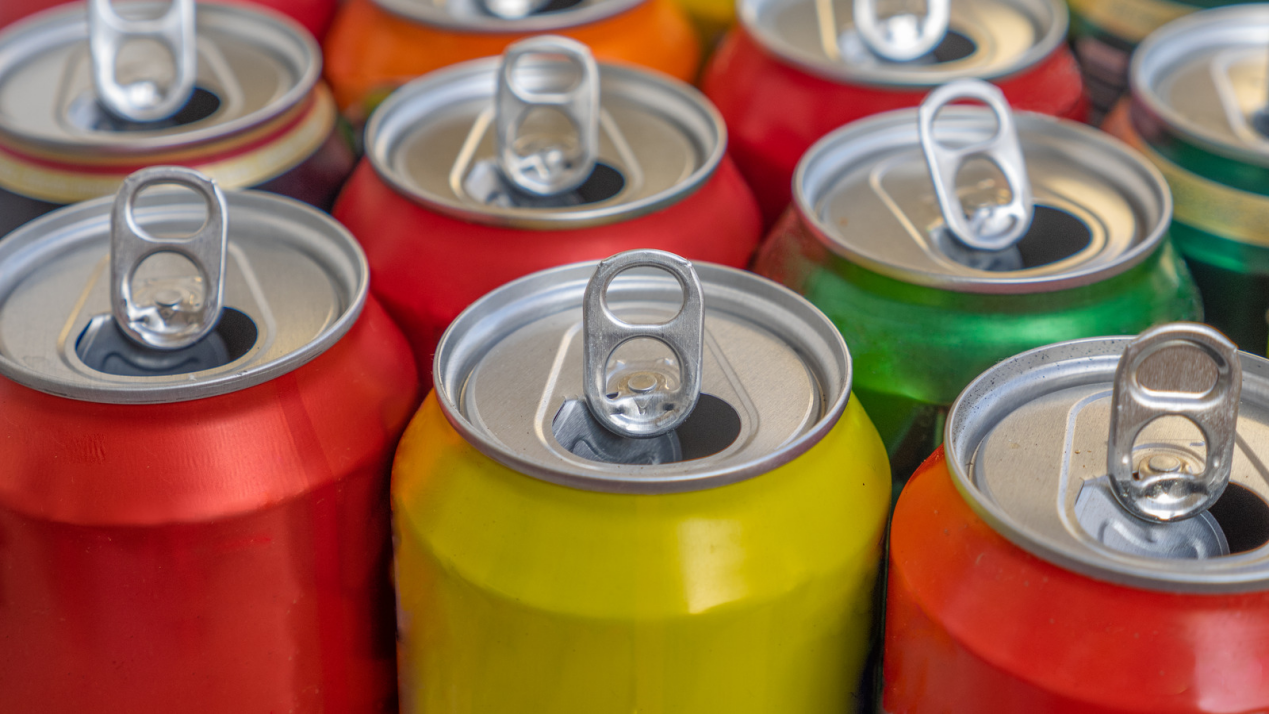
Leading aluminum companies in the production of aluminum strips for beverage cans, such as Alcoa in the United States, are aiming for thin walls of around 0.18mm. This development trend is also crucial for domestic aluminum strip manufacturers for beverage cans. They must increase their research and development efforts, adjust their technological research directions, and keep up with the global industry’s development to enhance competitiveness.
Research is underway to develop a single alloy to replace the original alloys such as 3104, 5182, and 5052, making it easier for management, production, and recycling.
Beverage cans are made from three different alloys (3004 for can bodies, 5182 for can lids, and 5042 for pull tabs). This presents challenges for recycling and remelting. Therefore, with the increasing environmental awareness, a unified alloy that integrates the can body, lid, and pull tab is a new direction for the development of aluminum used in beverage cans. Some known unified canning alloys include 5017 and 5349 from Golden Aluminum in the United States. Additionally, a Japanese patent, Tokkōshō 61-9180, describes a unified canning aluminum alloy containing 0.5%-2.0% Mn, 0.4%-2.0% Mg, 0.5% Si, 1.0% Fe, 0.5% Cu, 0.5% Zn, 0.2% Cr, 0.01% Be, 0.2% Ti, with the remainder being aluminum.
The United States is currently developing a 0.3mm thick can material and changing the shape of the cup bottom from circular to polygonal. It is said that this can significantly reduce the earing rate, resulting in more material savings than just thinning the material. This new invention is worth noting.
Due to the inability to reseal the lid once opened, traditional can lids have gradually been neglected. As a result, several Japanese companies have started using twist-off caps on beverage cans. This new type of beverage can provides better sealing, effectively preventing contact between the beverage and sunlight or oxygen. Additionally, it is lighter in weight and facilitates recycling and reuse. Currently, as this new type of beverage can is gradually gaining popularity in Japan, consumers are showing an increasing preference for twist-off caps. Can manufacturers in Japan hope to regain the market share that has been occupied by plastic bottles by using this product.
In addition to developing new alloys and varieties, can material processing technologies, such as hot and cold rolling and heat treatment, are also evolving towards wider and thinner dimensions. One of the characteristics required for aluminum alloy strips used for can lids is low anisotropy. To minimize fluctuations in the mechanical properties or formability of the strips, strict control of the alloy composition, as well as precise control of hot and cold rolling conditions and plate thickness deviations, is necessary.
In terms of processing methods, it has been reported that developed countries have started using continuous casting and rolling technologies to produce aluminum alloy materials for cans, further reducing processing costs. However, further verification is needed in this regard.
Southwest Aluminum (Group) Co., Ltd., the only domestic producer of aluminum materials for beverage cans, has achieved mass production of “ultra-thin aluminum sheets.” The historical monopoly of aluminum can materials by foreign countries in China no longer exists. Since 1986, Southwest Aluminum has organized a group of experts and engineering technicians to collaborate with relevant research institutes to develop technologies for producing aluminum materials for beverage cans. Over the past ten years, two generations of technical personnel have participated in the development of this product. With the strong support and impetus from national “Ninth Five-Year Plan” scientific and technological research projects, “863” research projects, and the technology development funds of China Aluminum Corporation, Southwest Aluminum has invested a significant amount of money to address the necessary equipment for can material production. They have also devoted substantial human and material resources to the development of can material production technology. Leveraging the advantages of advanced “1+1” hot rolling production line equipment and Southwest Aluminum’s technical expertise, they have optimized and innovated the can material production process and technology based on the characteristics of “1+4” hot continuous rolling. They have developed a complete set of new technologies suitable for advanced “1+4” equipment for can material production. Southwest Aluminum was the first to develop this product domestically and has gradually reduced the product thickness from the initial 0.42mm to 0.285mm and currently to 0.275mm, which is only one step away from the international advanced level of 0.265mm. The produced can materials meet high quality standards. According to industry experts, by the latest estimate of 2008, the competitive landscape of the global aluminum can material market is expected to change, and China has the potential to become one of the world’s six major producers of can materials.
Currently, there are eight countries in the world that can produce aluminum alloy can materials, including the United States, Brazil, Australia, Japan, South Korea, Germany, France, and Russia. Additionally, some countries and regions such as Taiwan Province of China, Canada, Bahrain, and Italy can produce can lid and pull-tab materials.
However, with the development of China Southwest Aluminum Co., Ltd. in can material production and the commissioning of the hot rolling production line by Nanshan Group Aluminum Processing Co., Ltd. earlier this year, as well as the hot rolling production line of Asia Aluminum Industrial Park that started operation by the end of 2007, and the joint trial run of China’s Bohai Aluminum Industry Co., Ltd. in 2008, the can materials produced in China will participate in the global can material market competition, and China has the possibility to become one of the world’s six major can material producers.
In 2008, the can material production of China Southwest Aluminum Co., Ltd. could reach 70,000 tons, while Nanshan Group and Asia Aluminum Group may produce around 30,000 tons. The total national production could reach about 100,000 tons, accounting for approximately 55% of the domestic demand. Due to the strong technical support from Alcoa, it is estimated that after the normal operation of equipment, China’s Bohai Aluminum Industry Co., Ltd. can produce competitive can materials in China and Southeast Asia within approximately 8 months. Its trial production time will be the shortest among the four major enterprises in China.
A key constraint factor that has hindered the localization of aluminum ingots for pressure purposes in the past is the neglect of effectively improving the metallurgical quality of aluminum materials. While strengthening purification and other molten metal treatments, it is also crucial to conduct in-depth and systematic research on the mechanisms of molten metal treatment and the plastic deformation behavior of aluminum materials. This provides a reliable theoretical basis and practical guidance for the rational development of cold and hot processing technology and effective control of product structure and performance.
Since aluminum ingots for pressure purposes must have excellent plastic deformation capability and a certain strength, it is important to study the influence of factors such as material chemical composition, rolling process, and annealing process on their mechanical properties and plastic deformation behavior. However, the current research in these areas is insufficient to further explore the performance potential of materials, especially in the research of low-grade materials and thin-walled cans. The influence of intrinsic metallurgical defects in materials becomes more prominent. Therefore, it is necessary to strengthen the comprehensive treatment of aluminum melt to fundamentally eliminate the main factors affecting the plastic deformation capability of aluminum materials.
Currently, researchers at Fuzhou University have conducted in-depth and systematic research and practical work on improving the metallurgical quality and plastic deformation performance of aluminum ingots. Particularly, they have proposed the purification principle of “priority to impurity removal and gas removal as a supplement” and the melt treatment principle of “purification as the basis for transformation and refinement.” Based on this, they have obtained efficient process technology for comprehensive treatment of aluminum melt and achieved significant progress in the research of low-grade materials and thin-walled cans, turning research results into productivity.